Molding and Casting
Molding and casting are manufacturing processes used to create multiple copies of a three-dimensional object. Here's an overview of each process: Molding: Molding involves creating a mold, which is a negative impression of the desired object. The mold is typically made from materials such as silicone rubber, urethane rubber, or other flexible materials. The process of creating the mold involves applying a mold release agent to the original object to prevent the mold material from sticking, then pouring or applying the mold material around the object. Once the mold material cures or solidifies, it is removed from the original object, leaving a cavity that matches its shape. Casting: Casting involves pouring a liquid material, such as plastic, metal, or resin, into the mold cavity created during the molding process. The liquid material is allowed to harden or cure within the mold, taking on the shape of the original object. Once the material has solidified, the mold is removed, and the newly formed object, known as the casting, is extracted. This casting is a replica of the original object, and multiple copies can be produced from the same mold. Molding and casting processes are widely used in various industries, including manufacturing, prototyping, and art and design. They offer several advantages, including the ability to create complex shapes and detailed replicas with relative ease and cost-effectiveness. Additionally, these processes allow for the production of multiple identical copies, making them suitable for mass production applications. However, it's essential to choose the appropriate materials and techniques for molding and casting based on the specific requirements of the project and the desired properties of the final products.
Stages of the process
Stage 1: idea
Stage 2: materials
Stage 3: result
1. Idea
This week I tried to make a film for a microfluidics device with natural polymers.
A microfluidic device is a small-scale system that manipulates and controls fluids at the microscale level, typically within channels or chambers with dimensions on the order of micrometers to millimeters. These devices are often fabricated using microfabrication techniques borrowed from the semiconductor industry, such as photolithography and soft lithography. Microfluidic devices offer precise control over the flow, mixing, and manipulation of fluids, and they find applications in various fields, including biology, chemistry, engineering, and medicine. Some common features and applications of microfluidic devices include: Lab-on-a-chip systems: Microfluidic devices can integrate multiple laboratory functions onto a single chip, enabling tasks such as sample preparation, analysis, and detection to be performed rapidly and with minimal sample volumes. Biomedical diagnostics: Microfluidic devices are used for point-of-care diagnostic tests, such as detecting pathogens, biomarkers, and genetic mutations. These devices can analyze small volumes of biological samples, offering fast and sensitive detection methods. Drug delivery systems: Microfluidic devices can be designed to precisely control the release of therapeutic agents, such as drugs or nanoparticles, at specific locations and rates within the body. This targeted drug delivery approach can improve treatment efficacy while minimizing side effects. Cell culture and tissue engineering: Microfluidic devices provide controlled microenvironments for culturing cells and tissues, allowing researchers to study cellular behavior, interactions, and responses under physiologically relevant conditions. These devices can also be used to create tissue constructs for regenerative medicine applications. Chemical synthesis and analysis: Microfluidic devices enable the synthesis of chemical compounds and the screening of reaction conditions on a small scale, leading to increased efficiency and reduced reagent consumption. They are also used for rapid chemical analysis, such as chromatography and spectroscopy. Overall, microfluidic devices offer versatile platforms for performing a wide range of tasks involving fluid manipulation and analysis, with potential applications across various scientific and technological disciplines.
2. Materials
Chitosan: Chitosan, a biocompatible and biodegradable polysaccharide derived from chitin, has been integrated into microfluidic devices for various applications due to its unique properties. Here are some ways chitosan has been used in microfluidic devices: Surface Modification: Chitosan can be used to modify the surface properties of microfluidic channels. By coating the channel walls with chitosan, researchers can alter the surface chemistry to control the flow behavior of fluids, enhance biocompatibility, and promote specific interactions with biomolecules or cells. Cell Culture and Tissue Engineering: Chitosan-based hydrogels have been incorporated into microfluidic devices to create microenvironments suitable for cell culture and tissue engineering applications. These hydrogels provide mechanical support and mimic the extracellular matrix, facilitating cell adhesion, proliferation, and differentiation within the microfluidic system.
Agar-agar: Agar agar, a gelatinous substance derived from seaweed, has been explored in microfluidic devices for various applications due to its biocompatibility, transparency, and ability to form stable gels at relatively low temperatures. Here are some ways agar agar has been integrated into microfluidic devices: Hydrogel Formation: Agar agar can be used to create hydrogel structures within microfluidic channels. By flowing an agar agar solution into microchannels and allowing it to gel, researchers can create stable three-dimensional structures that can mimic tissue environments or serve as scaffolds for cell culture and tissue engineering applications.
Alginate of sodium: utilized in microfluidic devices due to its unique properties, including biocompatibility, biodegradability, and the ability to form hydrogels in the presence of divalent cations such as calcium ions. Here are some ways sodium alginate has been integrated into microfluidic devices: Hydrogel Formation: Sodium alginate is commonly used to create hydrogel structures within microfluidic channels. By flowing an alginate solution into microchannels and subsequently introducing a solution containing calcium ions, researchers can trigger the gelation of sodium alginate, resulting in the formation of stable hydrogel structures. These alginate hydrogels can serve as scaffolds for cell encapsulation, tissue engineering, and drug delivery applications within the microfluidic platform.
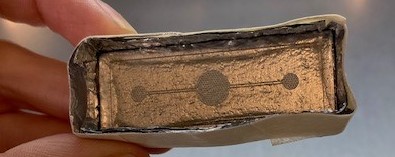
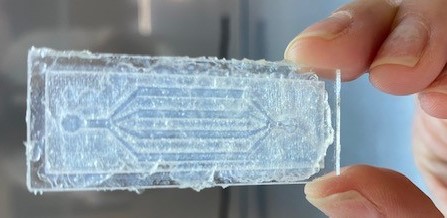
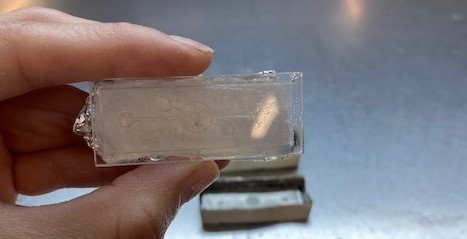
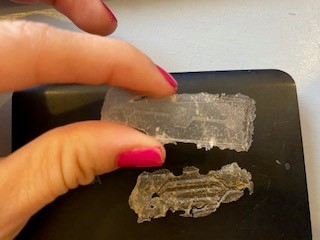
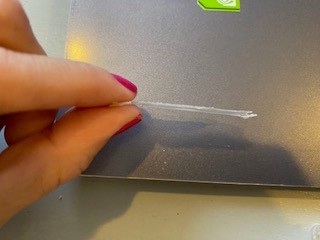
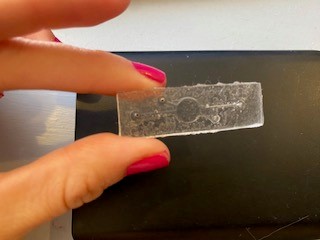
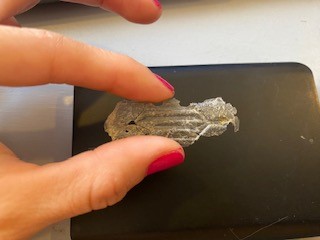
Conclusion
This week was not so complex This week I tested materials with which I had never developed a microfluidics device. I obtained a very thin, waterproof film which I can evaluate its resistance in cell growth evaluations. Better studies are required to evaluate the best drying temperature and time. The mixture of polymers made seems to be a good alternative for the bafrication of biofilms for microfluidic devices without the need for post-manufacturing biofunctionalization.